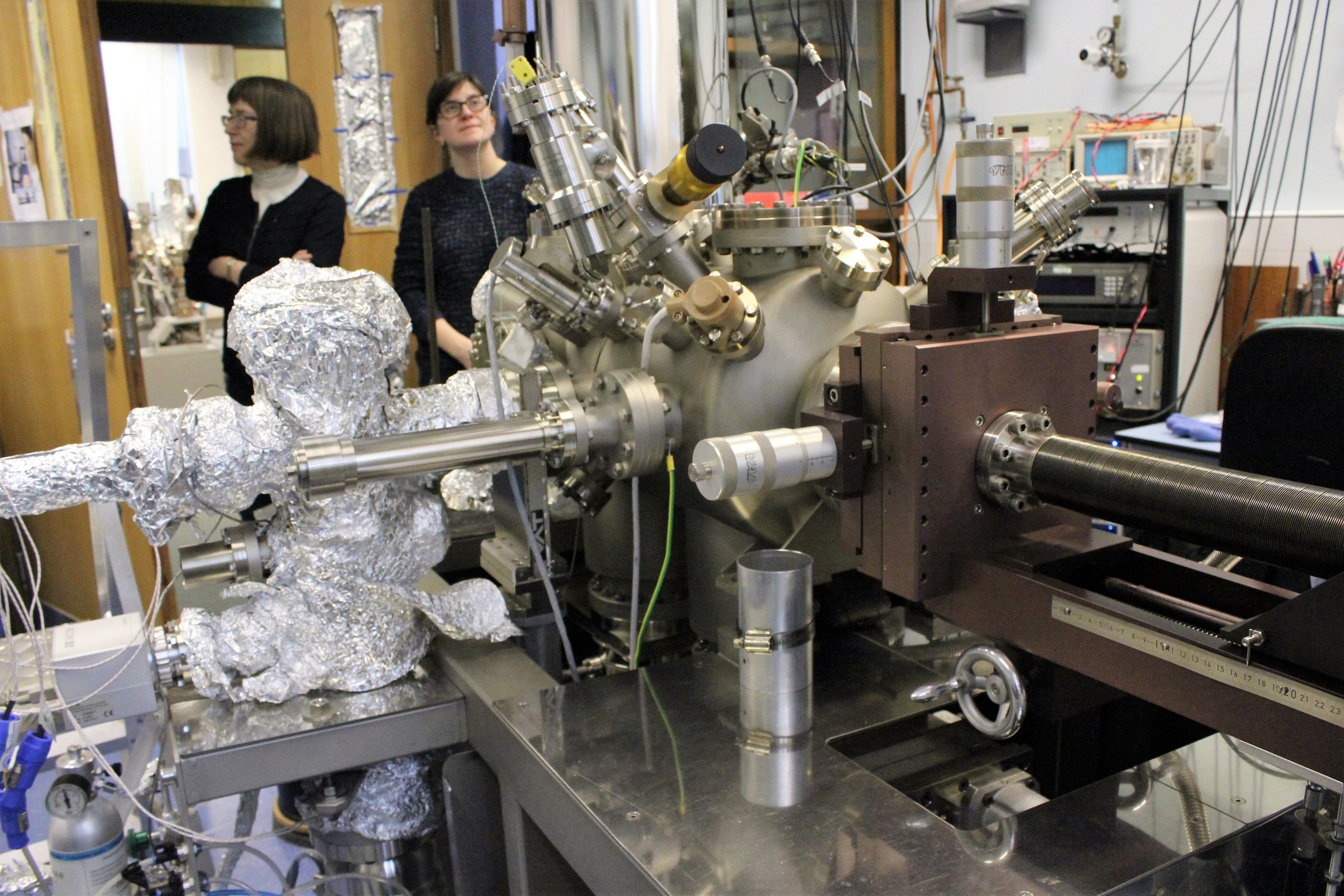
March 6, 2017, by Brigitte Nerlich
3D printing with atoms: Laboratory life
There is a long tradition of social scientists observing and analysing laboratory life. The most seminal book that has emerged from this tradition is probably Bruno Latour and Steve Woolgar’s 1979 Laboratory Life: The social construction of scientific facts (they changed the subtitle in 1986 to ‘the construction of scientific facts, as they became aware that ‘social’ was rather redundant in this context). Many more social science (Science and Technology Studies) books and articles followed.
Despite this, or because of this, ‘scientific facts’ have got a bit of a sociological and, more recently, political bashing and a lot of stereotypes about what science is and what facts are still circulate amongst science observers and critics of science. Philip Moriarty and I witnessed this yet again at a social science conference a few years ago. So when Phil wrote a grant application on 3D printing with atoms, he included as an aim: “an analysis of, and commentary on, the scientific method as practised in a nanoscience laboratory setting, i.e. to provide some insights into how science really happens”; and I came on board.
For Phil and myself it is important to see “science as a living and breathing thing, not as a body of facts in stasis”. In the interview from which this quote is taken, Phil goes on to say: “I’m a microscopist, image analysis is our stock-in-trade, and there’s definitely something very visceral about an image. However, physics is conceptually challenging, there’s an awful lot you really have to mull over for a [long time] before some of it clicks. We can, if we’re not careful, set up a culture whereby you watch this two- or three-minute video and think: I’m an expert. Thankfully, the vast majority of people don’t do that.”
In this post I will attempt to provide a brief glimpse into the messy and imperfect world of nanoscience and the hard work that goes into the construction of both facts and expertise. I also want to give a glimpse of the difficulties that emerge when talking across and between scientific specialisms and cultures.
The project and the team
I am involved in a project with Phil Moriarty and others for which the short-hand title is ‘3D printing with atoms’. I have reported on some outreach and science communication activities in other posts. In this one I want to focus not so much on how we are trying to communicate about this project with the outside world, but look instead at the complexities of communication within the world of the project – i.e. within the life of the lab.
The scientific team consists of three experimentalists, Philip Moriarty, Adam Sweetman and Filipe Junqueira, all working at the University of Nottingham; two theorists, David Abbasi Pérez and Lev Kantorovich at King’s College London; and me, the ‘social scientist’.
The scientific aim is to fabricate a 3D object – a nanoparticle – an atom at a time using a scanning probe microscope. Although there are now many examples of scientists being able to control single atoms in order to form a variety of artificial structures on surfaces, so far a 3D object has not been constructed atom by atom under computer control.
This construction project uses various types of scanning probe microscopes, which are quite different from normal optical microscopes (Phil has done many videos on this, one of the latest can be watched here – in the left hand corner you can see a model of gallium arsenide (GaAs) about which more later!).
As the word ‘scanning’ indicates, these microscopes, in this case an atomic force microscope, use a ‘tip’ to probe a surface (but without making contact with it). The (quantum-mechanical) signals that emerge from the interaction between the tip and the surface are sent to a computer and converted into images. The surface we are using at the moment is made of gallium arsenide (GaAS), a compound of the elements gallium and arsenic – and a semiconductor. The important thing in all this is the tip of the probe which ‘terminates’ in an atom and the relation between that atom and an atom or atoms directly underneath the tip or probe. By scanning the tip back and forth across the surface whilst monitoring how the chemical force changes, it is possible to build up an image of atoms. However, more importantly, we are using this same principle to try and build up something out of the atoms we are imaging.
Experimentation, simulation and visualisation
Reaching the project’s aim of building a structure out of atoms depends on the teamwork between what in the context of biology one would call the collaboration between ‘wet and dry‘ scientists. In biology a wet lab is a traditional laboratory, where chemicals, drugs, or other material or biological matter are handled, while a dry lab is a space where computer modellers generate descriptions of how certain biological processes would behave. In our case, the ‘wet’ people are what one can call the atomic force handlers (no squishiness though!) and the lab is full of complicated ‘probes’ (see featured images), and the ‘dry’ people are the theorists, modellers or simulators. While the experimentalists use as their working tools AFMs – atomic force microscopes – and DFMs – dynamic force microscopes, the theoreticians use numbers as their working tools, as well as DFT or density functional theory. This is a computational quantum mechanical modelling method (I had to laugh when I read this beginners guide to DFT!).
Both teams produce images. Experimentalists handle atomic forces to produce images. I wanted to say … to produce images of atoms. But that’s not quite true, as sometimes the white spots they observe are not atoms and they may not ‘see’ some atoms. Modellers meanwhile handle numbers to produce images. They simulate atoms and surfaces and produce images of particular arrangements of atoms. If experimentally derived images and the images produced through simulation coincide that means that the model is most likely correct and that the experimental images show what the team were trying to find.
Overall, the experimentalists look to the modellers to tell them what to look for and the modellers look to the experimentalists to tell them more about what they found and what to look for next and so on, in a sort of circular give and take between atomic manipulators and atomic modellers … until the results are agreed between them and the paper can be written.
Both teams speak ‘chemistry’ (and maths), which seems to be the bridging language between the modellers and the experimentalists, a language that, together with the language of maths and quantum stuff, I am still trying to understand.
The meeting
On 24 February, the whole team ‘met’ by Skype to look at some new images that Filipe and Adam had produced using an AFM (with Phil, Adam, Filipe and me clustered around Phil’s computer and David and Lev skyping in). There was some excitement about this meeting, as a month or so ago an accident in the lab had meant an imaging experiment had to be abandoned and redone. Phil’s email said: “Filipe and Adam have been getting some very nice atomic resolution NC-AFM [non-contact atomic force microscopy] images of GaAs(110) over the last week. … I know it’s very short notice but would you be free at 10:00 am this Friday for a Skype call to discuss progress with the project? “
Although the meeting had initially been called to look at Filipe and Adam’s images, we first looked at some images produced by David using theoretical simulation rather than physical scanning probes.
The tip and the termination
Figure 1: Simulated tip and surface interaction
The image we first looked at represented a ‘tip’ hovering over a surface and then crashing into it (something that is very important in this work, as ‘crashing’ is used to form a new tip). The tip was referred to in shorthand as a ‘tungsten’ tip and the surface was gallium arsenide. Although I first didn’t quite know what tungsten was (and if you don’t, watch this video!), it turned out to be a metal and the discussion turned around other metals that could be used as the basis for the tip, such as gold, sliver or silicon. There was a lot of discussion about the ‘tip’ (“a proper tip”, “a perfect tip”) and so on. In the end Adam suggested that gold might be better than tungsten.
When listening to the discussion as a non-nanoscientist, I was getting confused between what people called the ‘tip’ and the ‘termination’. Interestingly, when discussing the image of the simulation, one of the experimentalists noted that the “atom on the end will not be happy; it’ll want to go nuts”. The ‘happiness’ of the atom seemed to depend on the tip/termination. My own ‘happiness’, I began to think, depended largely on finding out what the relation was between a tip, a termination and a surface – and atoms, of course!
When I asked Phil afterwards on the way to tea about this, he quickly picked up a gallium arsenide model, pointed to it and said this was the termination. When I asked where the gold was, he stretched out his arm and pointed into space saying “somewhere over there” – hmmm, obviously. I should have resumed that conversation after tea! In the end I did it through email. I got two explanations about my tip mystery, one from Phil and one from Filipe.
Phil said: “The GaAs cluster is stuck to the end of a gold probe (or, more correctly, a gold-coated probe that started off being terminated in tungsten oxide, most likely). We take a tungsten wire and etch it electrochemically to a sharp point. The etching process dissolves away tungsten. When we introduce the tip to the microscope initially it’s almost certainly terminated by a tungsten oxide layer because it’s been etched and then transferred through air to be put in a chamber out of which we then pump the air.”
Filipe said: “The discussion about what the tip was made of was actually a discussion of what the last atomic layers (closer to the apex of the tip) are mostly made of. We have a tip that is made of a tungsten wire. But because we have crashed the tip quite heavily on silver, we can expect the last atomic layers of the tip to be made of silver. And as far as DFT is concerned, we only care about the last layers. The conclusion of the discussion we had was that we should prepare our tip into gold directly, instead of silver. Therefore it would make more sense for Lev and David to model the tip being made out of gold, instead of silver or tungsten.” That’ll take a bit more time.
Dots, spots and lines
We then finally looked at Adam and Filipe’s slides of the images produced using an AFM. These were “the best images from the last run”. There was some debate about the scale – was it 15 nanometers or not? Then the discussion became more interesting for me, as words were being used that I could understand 😉 They talked about how to interpret the “dark patches” – but here a word was used I didn’t get, what I transcribed in my notes as ‘dopends’. I had to email Phil about that. His reply was: “That’s my accent again! It’s ‘dopants’. They’re impurity atoms that are added to a semiconductor material to change its electrical resistance. A dopant atom either has one more, or one less, valence electron than its ‘host’ atoms.” I never would’ve guessed! If you want to know more, here is a good explanation”. So, ‘dark patches’ have a much deeper meaning, of course, than I imagined. The same probably applies in spades for the other words I picked out of the conversation! As I once declared, I tend to paddle in the shallow end of (this type of) knowledge!
When looking at the Powerpoint slides, the Nottingham scientists used words like “steppy”, “terrace”, “rows” that change direction. When looking at slide 10, they chatted about “rows” again but also about a “big spot”, a “square” and something being “Ziggy zaggy”.
Figure 2: Slide from a powerpoint presentation showing AFM-based images
For me the most intriguing exchange occurred around slide 16, when the Phil turned to me and said this was a bit like art and they had no idea how to interpret what they saw. They thought it was “funny” and asked “Where are the atoms?” and then concluded “We don’t know”. This reminded me of Catherine Allamel-Raffin’s analysis of shop-talk around a microscope and the way that scientists interpret images using ‘techniques’ that we also use when interpreting art.
Figure 3: Powerpoint slide showing puzzling images
Phil then picked up the physical model of gallium arsenide so that they could compare it with the images on the computer screen and they continued their discussion.
Figure 4: Phil holding model of GaAS
Conclusion
Back to Latour and Woolgar. In their classical study of laboratory life, they say that “[o]ne way to examine the microprocesses of fact construction in science is by looking at conversation and discussions between members of the laboratory” (1986[1979]: 154). After observing various conversation in their lab (the Salk Institute), they produced an interesting diagram representing “the different preoccupations of the conversations” they observed.
Listening to Phil and the team talking and trying to interpret various images in order to get, in the end, an article published with reproducible results, I could see that this type of talk and the dynamics of the conversation I overheard maps quite nicely onto this diagram.*
And finally: When emailing forwards and backwards about a draft of this blog post, it became apparent that my naive descriptions of our conversation had an interesting defamiliarisation effect for the natural scientists and also stimulated some discussion among the team. One team member remarked: “Amazing how things so obvious to us sound so futuristic to non-specialists”. And one of the experimentalists asked the simulators whether he was talking ‘gibberish’. I felt better after that.
Footnote:
The figure is figure 4.1 in the blog post to which I hyperlinked. I didn’t know whether there were copyright issues attached to reproducing the figure or not, so I didn’t do that for my own post. In this footnote I want to guide the reader to one important sentence in the blog post that uses the figure: “The current widespread ‘science is social hence it’s a bunch of hot air’ notion simply isn’t in the book at all, nor does it follow from anything they report or say.” That’s important to keep in mind!
And here are some quotes from Laboratory Life that dispel any ‘relativist’ notions: “To say that [a particular hormone] is constructed is not to deny its solidity as a fact. Rather, it is to emphasize how, where and why it was created.” (p. 127) And: “We do not wish to say that facts do not exist nor that there is no such thing as reality. In this simple sense we are not relativist. Our point is that ‘out-there-ness’ is the consequence of scientific work rather than its cause.” (pp. 181-182)
Image: Shey and Brigitte in the lab
No comments yet, fill out a comment to be the first
Leave a Reply