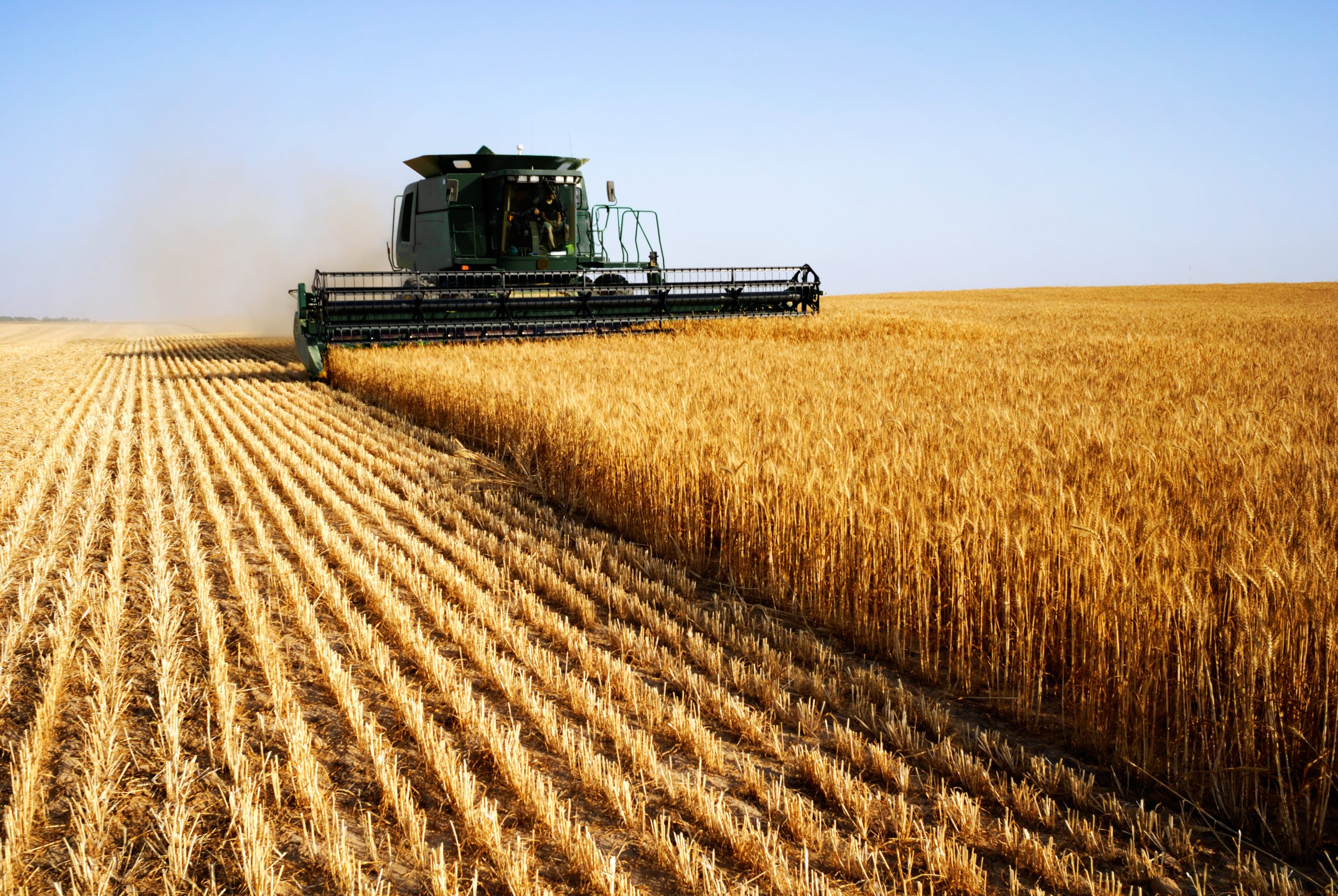
January 24, 2023, by Lexi Earl
Disease susceptibility and genes in wheat
Last week we explored the ways scientists are working to diversify wheat genes to combat vulnerabilities to disease. This week, PhD candidate Nicola Walter takes us on a deep dive into gametocidal genes – selfish elements that preferentially transmit themselves to new plants by destroying those chromosomes that don’t have the gene.
Gametocidal genes
Genetic diversity in crops is incredibly important to prevent susceptibility to disease. The King’s group at BBSRC Nottingham Wheat Research Centre, based at University of Nottingham, are using wild relatives of wheat as a novel source of genes to breed in disease resistance, among other useful traits such as high protein content and salt tolerance in our modern varieties. However, as with most science, this is not quite as simple as it sounds.
One issue (of many) that challenges breeding modern wheat with wild relatives is gametocidal genes, also known as “cuckoo” chromosomes. These are selfish elements that preferentially transmit themselves to offspring by destroying the chromosomes in the other gametes (pollen and eggs) that don’t contain the gene. An analogy is its namesake, the cuckoo. A cuckoo bird lays an egg in another bird’s nest, tricking the other bird into raising the cuckoo’s young. However, once the chick hatches, it shoves all the other eggs out of the nest, ensuring its own survival in place of the others.
The problem that these genes present is that if half of your gametes have the gene and half do not, only half will turn to seed, which results in less grain. And because these genes preferentially transmit to the next generation, we can’t use traditional breeding techniques such as selection to remove them, because all the offspring have the gene. To add insult to injury, seeds tend to be shrivelled, inviable and the plants are unhealthy.

An example of shrivelled seeds (below) as compared to healthy ones.
A theory for how gametocidal genes work is the dual-mechanism model: the ‘breaker’ and ‘inhibitor’. The breaker element sends out proteins that cause breakage in chromosomes, unless the ‘inhibitor’ element has protected the chromosomes from the breakage. Thus far, the mechanism behind this has still not been discovered, though theories involving epigenetics and small RNA molecules have been mentioned.
There have been suggestions that the gametocidal dual-mechanism model could involve transposons similar to that of fruit flies. Transposons, or “jumping genes”, are small pieces of DNA that can cut-and-paste themselves from one area of the genome into another. Fruit flies have a type of transposon in germline cells called P-elements, which induce identical symptoms to gametocidal action, such as sterility, chromosomal breakage and mutations that can lead to abortion of the gametes. There are two types of flies, P-strain and M-strain. P-strain flies possess the P-elements. When a male P-strain mates with a female M-strain, hybrid dysgenesis occurs whereby the P-element can transpose, or move, to different locations in the genome of germline cells potentially becoming mutagenic if it lands within a gene. However, when a male M-strain mates with a female P-strain, hybrid dysgenesis does not occur. This is because there is a high concentration of P-element repressor molecules active in P-strain eggs that prevent the transcription of transposase, the enzyme needed for transposition.
Similarities between the dual-mechanism model and a restriction-modification system found in bacteria have also been recognised. In this system, restriction enzymes are produced that cleave a specific site in the genome, unless the sites are methylated by the modification enzymes. Nonetheless, these theories are still speculative and evidence is still lacking to explain exactly how these menacing genes work. Despite this, efforts can still be made to remove them.
Removing the gene
There are many wild relatives currently being used to introduce novel genes with useful traits into wheat. Unfortunately, a number of these also contain gametocidal genes. This means that whilst many wheat-wild relative lines with incredibly useful traits such as rust resistance and increased protein content have been made, they cannot be sent to breeders as the gametocidal gene is also transmitted, causing the line to be agronomically unsuitable because of the low seed set, shrivelled seeds and unhealthy plants.
Since we can’t use traditional breeding techniques in this case, other methods are being developed. One has been to use a non-GM chemical mutagen on a wheat-wild relative introgression line containing a gametocidal gene to try and render the ‘breaker’ element non-functional. Through crossing this mutated line with a gametocidal line, all the gametes would remain protected by the still functional ‘inhibitor’ elements. There would be no preferential transmission and we can select for plants that only contain the broken gene. In the subsequent generations, the gene can be removed entirely.
Opportunities
In science, problems can sometimes be opportunities in disguise. What would happen if we took this pesky gene that preferentially transmits itself and attached a useful one to it? In the 1990s, Prof. Ian King did exactly that, ensuring that a gene from a wild relative improving flour quality remained within the wheat through generations.
We have not yet found the gene responsible for gametocidal action. However, with so many potentially useful wheat-wild relative lines waiting to have their gametocidal genes removed, once mutants are developed the impact will be high – having a knock-on effect for food security globally. And what is a better motivation than improving food security?
No comments yet, fill out a comment to be the first
Leave a Reply